Abstract
The classic mode of STING activation is through binding the cyclic dinucleotide 2′3′-cyclic GMP–AMP (cGAMP), produced by the DNA sensor cyclic GMP–AMP synthase (cGAS), which is important for the innate immune response to microbial infection and autoimmune disease. Modes of STING activation that are independent of cGAS are much less well understood. Here, through a spatiotemporally resolved proximity labelling screen followed by quantitative proteomics, we identify the lysosomal membrane protein Niemann–Pick type C1 (NPC1) as a cofactor in the trafficking of STING. NPC1 interacts with STING and recruits it to the lysosome for degradation in both human and mouse cells. Notably, we find that knockout of Npc1 ‘primes’ STING signalling by physically linking or ‘tethering’ STING to SREBP2 trafficking. Loss of NPC1 protein also ‘boosts’ STING signalling by blocking lysosomal degradation. Both priming and boosting of STING signalling are required for severe neurological disease in the Npc1−/− mouse. Genetic deletion of Sting1 (the gene that encodes STING) or Irf3, but not that of Cgas, significantly reduced the activation of microglia and relieved the loss of Purkinje neurons in the cerebellum of Npc1−/− mice, leading to improved motor function. Our study identifies a cGAS- and cGAMP-independent mode of STING activation that affects neuropathology and provides a therapeutic target for the treatment of Niemann–Pick disease type C.
Access options
Subscribe to Journal
Get full journal access for 1 year
199,00 €
only 3,90 € per issue
Tax calculation will be finalised during checkout.
Rent or Buy article
Get time limited or full article access on ReadCube.
from$8.99
All prices are NET prices.
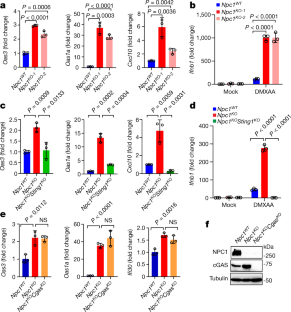
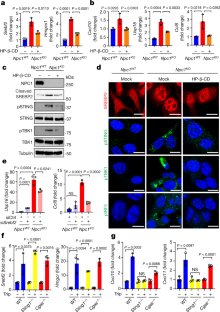
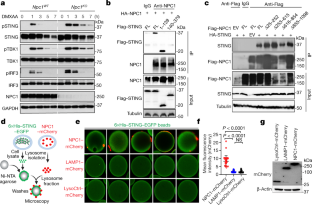
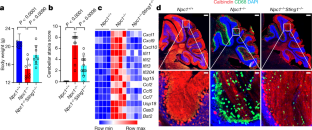

Data availability
There are no restrictions on data availability in this manuscript. All of the information is included in the manuscript. Original western blots and TMT-MS raw data are included as Supplementary Fig. 1 and Supplementary Data, respectively.
References
- 1.
Chin, A. C. Neuroinflammation and the cGAS–STING pathway. J. Neurophysiol. 121, 1087–1091 (2019).
- 2.
Yan, N. Immune diseases associated with TREX1 and STING dysfunction. J. Interferon Cytokine Res. 37, 198–206 (2017).
- 3.
Sliter, D. A. et al. Parkin and PINK1 mitigate STING-induced inflammation. Nature 561, 258–262 (2018).
- 4.
Yang, K., Huang, R., Fujihira, H., Suzuki, T. & Yan, N. N-glycanase NGLY1 regulates mitochondrial homeostasis and inflammation through NRF1. J. Exp. Med. 215, 2600–2616 (2018).
- 5.
Yu, C.-H. et al. TDP-43 triggers mitochondrial DNA release via mPTP to activate cGAS/STING in ALS. Cell 183, 636–649 (2020).
- 6.
Wu, J. et al. STING-mediated disruption of calcium homeostasis chronically activates ER stress and primes T cell death. J. Exp. Med. 216, 867–883 (2019).
- 7.
Warner, J. D. et al. STING-associated vasculopathy develops independently of IRF3 in mice. J. Exp. Med. 214, 3279–3292 (2017).
- 8.
Luksch, H. et al. STING-associated lung disease in mice relies on T cells but not type I interferon. J. Allergy Clin. Immunol. 144, 254–266.e8 (2019).
- 9.
Motwani, M. et al. Hierarchy of clinical manifestations in SAVI N153S and V154M mouse models. Proc. Natl Acad. Sci. USA 116, 7941–7950 (2019).
- 10.
Dobbs, N. et al. STING activation by translocation from the ER is associated with infection and autoinflammatory disease. Cell Host Microbe 18, 157–168 (2015).
- 11.
Barber, G. N. STING-dependent signaling. Nat. Immunol. 12, 929–930 (2011).
- 12.
Wu, J., Dobbs, N., Yang, K. & Yan, N. Interferon-independent activities of mammalian STING mediate antiviral response and tumor immune evasion. Immunity 53, 115–126 (2020).
- 13.
Gonugunta, V. K. et al. Trafficking-mediated STING degradation requires sorting to acidified endolysosomes and can be targeted to enhance anti-tumor response. Cell Rep. 21, 3234–3242 (2017).
- 14.
Pokatayev, V. et al. Homeostatic regulation of STING protein at the resting state by stabilizer TOLLIP. Nat. Immunol. 21, 158–167 (2020).
- 15.
Hung, V. et al. Spatially resolved proteomic mapping in living cells with the engineered peroxidase APEX2. Nat. Protoc. 11, 456–475 (2016).
- 16.
Gui, X. et al. Autophagy induction via STING trafficking is a primordial function of the cGAS pathway. Nature 567, 262–266 (2019).
- 17.
Srikanth, S. et al. The Ca2+ sensor STIM1 regulates the type I interferon response by retaining the signaling adaptor STING at the endoplasmic reticulum. Nat. Immunol. 20, 152–162 (2019).
- 18.
Brown, M. S., Radhakrishnan, A. & Goldstein, J. L. Retrospective on cholesterol homeostasis: the central role of Scap. Annu. Rev. Biochem. 87, 783–807 (2018).
- 19.
Chen, W. et al. ER adaptor SCAP translocates and recruits IRF3 to perinuclear microsome induced by cytosolic microbial DNAs. PLoS Pathog. 12, e1005462 (2016).
- 20.
Taylor, A. M., Liu, B., Mari, Y., Liu, B. & Repa, J. J. Cyclodextrin mediates rapid changes in lipid balance in Npc1−/− mice without carrying cholesterol through the bloodstream. J. Lipid Res. 53, 2331–2342 (2012).
- 21.
Duncan, E. A., Brown, M. S., Goldstein, J. L. & Sakai, J. Cleavage site for sterol-regulated protease localized to a Leu–Ser bond in the lumenal loop of sterol regulatory element-binding protein-2. J. Biol. Chem. 272, 12778–12785 (1997).
- 22.
Hua, X. et al. SREBP-2, a second basic-helix-loop-helix-leucine zipper protein that stimulates transcription by binding to a sterol regulatory element. Proc. Natl Acad. Sci. USA 90, 11603–11607 (1993).
- 23.
Li, X. et al. Structure of human Niemann–Pick C1 protein. Proc. Natl Acad. Sci. USA 113, 8212–8217 (2016).
- 24.
Chu, B.-B. et al. Cholesterol transport through lysosome–peroxisome membrane contacts. Cell 161, 291–306 (2015).
- 25.
Fog, C. K. & Kirkegaard, T. Animal models for Niemann–Pick type C: implications for drug discovery & development. Expert Opin. Drug Discov. 14, 499–509 (2019).
- 26.
Elrick, M. J. et al. Conditional Niemann–Pick C mice demonstrate cell autonomous Purkinje cell neurodegeneration. Hum. Mol. Genet. 19, 837–847 (2010).
- 27.
Guyenet, S. J. et al. A simple composite phenotype scoring system for evaluating mouse models of cerebellar ataxia. J. Vis. Exp. 39, e1787 (2010).
- 28.
Shin, S. D. K. et al. Interferon downstream signaling is activated early in pre-symptomatic Niemann–Pick disease type C. Neurosci. Lett. 706, 43–50 (2019).
- 29.
Parra, J. et al. Npc1 deficiency in the C57BL/6J genetic background enhances Niemann–Pick disease type C spleen pathology. Biochem. Biophys. Res. Commun. 413, 400–406 (2011).
- 30.
Cougnoux, A. et al. Microglia activation in Niemann–Pick disease, type C1 is amendable to therapeutic intervention. Hum. Mol. Genet. 27, 2076–2089 (2018).
- 31.
Sleat, D. E. et al. Genetic evidence for nonredundant functional cooperativity between NPC1 and NPC2 in lipid transport. Proc. Natl Acad. Sci. USA 101, 5886–5891 (2004).
- 32.
Dixit, S. S. et al. Loss of Niemann–Pick C1 or C2 protein results in similar biochemical changes suggesting that these proteins function in a common lysosomal pathway. PLoS ONE 6, e23677 (2011).
- 33.
Decout, A., Katz, J. D., Venkatraman, S. & Ablasser, A. The cGAS–STING pathway as a therapeutic target in inflammatory diseases. Nat. Rev. Immunol. https://doi.org/10.1038/s41577-021-00524-z (2021).
Acknowledgements
We thank G. Barber for Sting1−/− mice, K. Fitzgerald and T. Taniguchi for Irf3−/− mice, Z. Chen for Cgas−/− and Mavs−/− mice and J. Dietschy for Npc2+/− mice; D. Ramirez, W. Li and A. Nawaby at the University of Texas Southwestern (UTSW) Whole Brain Microscopy Core Facility (RRID:SCR_017949) for assistance with tissue sectioning, histological staining, immunostaining and slide scanning; A. Lemoff at the UTSW Proteomics Core Facility for assistance with TMT-MS experiments; R. Hammer and M. Nguyen at the UTSW Transgenic Technology Center for creating Npc1−/−Sting1−/− mice; K. Phelps and A. Bugde at the UTSW Live Cell Imaging Facility, a Shared Resource of the Harold C. Simmons Cancer Center, supported in part by NCI Cancer Center Support Grant 1P30 CA142543-01 and National Institutes of Health (NIH) Shared Instrumentation Award 1S10 OD021684-01; I. Raman at the UTSW Medical Center Genomics and Microarray Core; and P. Tsai and members of the Yan laboratory for discussions. This work was supported by the NIH (AI151708 and NS122825 to N.Y.), the Cancer Prevention and Research Institute of Texas (CPRIT; RP180288 to N.Y.), the Burroughs Wellcome Fund (N.Y.) and the Ara Parseghian Medical Research Foundation (J.J.R.).
Author information
Affiliations
Contributions
T.-T.C. and N.Y. conceived and designed the study. T.-T.C. performed most of the experiments. X.T. helped with siRNA validation of the primary proteomic screen and in vitro experiments. K.Y. and J.W. helped with in vitro experiments. J.J.R. provided BALB/c wild-type, Npc1−/− and Npc2−/− mice and provided reagents. T.-T.C. and N.Y. wrote the paper with inputs from all co-authors.
Corresponding author
Ethics declarations
Competing interests
The authors declare no competing interests.
Additional information
Peer review information Nature thanks Veit Hornung and the other, anonymous, reviewer(s) for their contribution to the peer review of this work. Peer reviewer reports are available.
Extended data figures and tables
Extended Data Fig. 1 Screen of STING trafficking cofactors using spatiotemporally resolved proximity labelling and quantitative proteomics.
a, Diagram for proximity labelling and proteomic discovery of trafficking cofactors using Sting1−/− MEFs stably expressing STING–APEX2. b, Representative microscopy images of STING–APEX2 trafficking at various time points after stimulation (HT-DNA, 1 μg ml−1). BFA blocks the exit of STING from the ER; BafA1 blocks the degradation of STING by lysosomes. Scale bars, 10 μm. c, Immunoblot analysis of cell lysates. STING–APEX2 MEFs were mock-treated or stimulated with HT-DNA (1 μg ml−1) for the indicated times with or without treatment with BFA or BafA1 (top). Then, proximity labelling was performed and biotinylated proteins were detected by streptavidin–HRP. Immunoblot is representative of at least three independent experiments. d, TMT-MS quantitative proteomics data filtering from two independent experiments and candidate discovery. e, Diagram of the STING trafficking route after stimulation, including time points (top) and organelles. f, Heat map showing selected STING trafficking cofactor candidates at each organelle (top). MS value was normalized to the 0-h time point. Data are representative of three independent experiments.
Extended Data Fig. 2 NPC1 deficiency primes STING trafficking and activation independently of cGAMP.
a, qRT–PCR analysis of the baseline expression of ISGs (Oas3, Oas1a and Usp18) in Npc1WT, Npc1KO and Npc1KO MEFs stably expressing wild-type Npc1 (n = 4). b, qRT–PCR analysis of Ifnb1 mRNA expression in wild-type and Npc1-knockdown MEFs after stimulation with an increasing amount of Poly I:C (0, 1, 2, 4 μg ml−1) for 3 h (n = 3). c, Immunoblot analysis of the indicated proteins (left) in Npc1WT, Npc1KO and Npc1KOSting1KO MEFs. d, Heat map showing the baseline expression of ISGs in Npc1WT, Npc1KO and Npc1KOSting1KO MEFs reconstituted with vector, STING wild type (FL), R232A or S366A mutants (n = 2). The mRNA expression of each ISG was measured by qRT–PCR. e, Immunoblot analysis of the indicated proteins (left) in different reconstituted MEFs (shown on top) as in d. f, Fluorescent microscopy analysis of endogenous STING localization at the resting state in Npc1WT and two independent clones of Npc1KO MEFs. Endogenous STING is shown in green, an ERGIC marker (ERGIC53) is shown in red and DAPI is shown in blue. Scale bars, 10 μm. g, Quantification of STING co-localization with the ERGIC in f (Npc1WT, n = 13; Npc1KO-1, n = 12; Npc1KO-2, n = 13). h, qRT–PCR analysis of the baseline expression of ISGs in fibroblasts from healthy control individuals (n = 2) and unrelated patients with Niemann–Pick disease type C (NPC1I1061T) (n = 4). a, b, g, Data are mean ± s.d. a, g, Unpaired two-tailed Student’s t-test. Data are representative of at least two independent experiments.
Extended Data Fig. 3 SREBP2 trafficking primes STING signalling in Npc1KO cells independently of its transcriptional activity.
a, Diagram showing mechanisms of action for HP-β-CD and triparanol on cholesterol synthesis and SREBP2 activation. HP-β-CD promotes the egress of lysosomal cholesterol to the ER, thus limiting SREBP2 trafficking and activation in Npc1KO cells. Triparanol inhibits the biochemical conversion of desmosterol into cholesterol, thus promoting SREBP2 trafficking and activation in wild-type cells. b, c, qRT–PCR analysis of cholesterol-synthesis genes (b) and ISGs (c) in Npc1WT and Npc1KO MEFs mock-treated or treated with HP-β-CD (0.3 mM) overnight (n = 3). d, e, qRT–PCR analysis of mock-, DMXAA- (50 μg ml−1, 2 h) or poly(I:C)- (1 μg ml−1, 2 h) induced expression of cholesterol-synthesis genes (d) and ISGs (e) with mock- or HP-β-CD treatment (0.3 mM, 8 h) in Npc1WT MEFs (n = 3). f, qRT–PCR analysis of the baseline expression of ISGs in Npc1WT and Npc1KO MEFs transfected with siCtrl or siSrebf2 for 48 h (n = 3). g, Immunoblot analysis of the indicated proteins (left) in Npc1WT, Npc1KO, Npc1KOSrebf2KD and Npc1KOSrebf2KD MEFs reconstituted with SREBP2 wild type (FL) or transcription-inactive mutants (L511A/S512A, ΔbHLH). h, i, qRT–PCR analysis of the expression of cholesterol-synthesis genes (h) and ISGs (i) in Npc1WT, Npc1KO, Npc1KOSrebf2KD and Npc1KOSrebf2KD MEFs reconstituted with SREBP2 wild type (FL) or transcription-inactive mutants (L511A/S512A, ΔbHLH) (n = 3). b–f, h, i, Data are mean ± s.d. Unpaired two-tailed Student’s t-test. NS, not significant. Data are representative of at least two independent experiments.
Extended Data Fig. 4 SREBP2 primes STING signalling independently of cGAS or MAVS.
a, Immunoblot analysis of the SREBP2 and STING activation in Npc1WT MEFs that were treated with mock or triparanol (14 μM) for the indicated times (shown on the top). SREBP2 cleavage indicates activation. STING activation induces pSTING and pTBK1. b, Fluorescent microscopy analysis of SREBP2 and STING activation. Cleaved SREBP2 translocates to the nucleus (red). The STING activation markers pSTING, pTBK1 and pIRF3 are shown in green. The nucleus dye DAPI is shown in blue. Scale bars, 10 μm. c, qRT–PCR analysis of cholesterol-synthesis genes in BMDMs that were either mock-treated or treated with triparanol (14 μM) for 12 h (n = 3). d, qRT–PCR analysis of the baseline expression of ISGs in BMDMs that were either mock-treated or treated with triparanol (14 μM) alone or in combination with the STING inhibitor C-176 (0.5 μM) for 12 h (n = 3). e, f, qRT–PCR analysis of cholesterol-synthesis genes (e) and ISGs (f) in wild-type, Sting1−/− and Cgas−/− BMDMs that were either mock-treated or treated with triparanol (14 μM) for 12 h (n = 3). g, h, qRT–PCR analysis of the expression of cholesterol-synthesis genes (g) and ISGs (h) in Mavs−/− MEFs that were either mock-treated or treated with triparanol (14 μM) for 12 h (n = 3). i, j, qRT–PCR analysis of the cholesterol-synthesis gene Hmgcs1, knockdown efficiency of Insig1 (i) and the expression of ISGs (j) in wild-type, Cgas−/− and Sting1−/− MEFs (n = 3). c–j, Data are mean ± s.d. Unpaired two-tailed Student’s t-test. Data are representative of at least two independent experiments.
Extended Data Fig. 5 NPC1 interacts with STING and mediates the lysosomal degradation of STING.
a, Quantification of immunoblots in Fig. 3a. b, Immunoblot analysis of STING degradation in Npc1WT, Npc1KO or Npc1KO MEFs stably expressing wild-type NPC1. Cells were mock-treated or stimulated with HT-DNA (1 μg ml−1) for 8 h. c, d, Live-cell microscopy images of STING–EGFP and lysosomes. Npc1WT and Npc1KO MEFs stably expressing STING–EGFP were mock-treated (left) or stimulated with HT-DNA (right, 4 μg ml–1). STING–EGFP is shown in green and LysoTracker-Red is shown in red. Scale bar, 10 μm. Representative images in c and quantifications in d. Npc1WT 0 h (n = 13), Npc1KO 0 h (n = 17), Npc1WT 15 h (n = 15), and Npc1KO 15 h (n = 16) for quantification. Data are mean ± s.d. Unpaired two-tailed Student’s t-test. e, Immunoblot analysis of NPC1 and STING co-immunoprecipitation in HEK293T cells. HEK293T cells were transfected with the indicated plasmids (top), and 24 h later, anti-IgG (mouse) or anti-Flag was used for the pull-down. HA–STING co-immunoprecipitation was analysed by anti-STING immunoblot. Whole-cell lysates were blotted by anti-Flag (NPC1), anti-STING and anti-tubulin as input. f, Immunoblot analysis of endogenous STING and NPC1 co-immunoprecipitation in wild-type MEFs. Anti-IgG (rabbit) or anti-NPC1 (rabbit) was used for the pull-down. Both immunoprecipitation and lysate were blotted for endogenous STING (rabbit), NPC1 (mouse) and tubulin. g, Immunoblot analysis of endogenous STING and NPC1 co-immunoprecipitation in wild-type MEFs with mock-treated, DMXAA (30 μg ml−1, 8 h)-treated or DMXAA combined with BafA1-treated (to prevent STING degradation). Anti-IgG (rabbit) or Anti-NPC1 (rabbit) was used for the pull-down. Both immunoprecipitation and lysate input were blotted for endogenous STING (rabbit) and NPC1 (mouse). h, Immunoblot analysis of endogenous STING interaction with NPC1 or LAMP1 in wild-type MEFs. Anti-IgG (rabbit) or anti-STING (rabbit) was used for the pull-down. Both immunoprecipitation and lysate input were blotted for endogenous STING, NPC1 or LAMP1. Red arrow, co-immunoprecipitated NPC1 band. i, Live-cell fluorescent microscopy analysis of STING–EGFP and NPC1–mCherry localization. Npc1KOSting1KO MEFs stably expressing STING–EGFP (green) and NPC1–mCherry (red) were stimulated with HT-DNA (4 μg ml−1) and imaged at the indicated times. Scale bars, 10 μm. j, k, Diagrams showing STING (j) and NPC1 (k) full length and truncations. l, Immunoblot analysis of STING degradation in Npc1WT, Npc1KO or Npc1KO MEFs stably expressing indicated NPC1 truncations. Cells were mock-treated or stimulated with HT-DNA (1 μg ml−1) for 8 h. Cell lysates were analysed for the proteins indicated on the left. m, Immunoblot analysis of STING interaction with NPC1 transmembrane bundles in HEK293T cells. HEK293T cells were transfected with the indicated plasmids (top), and 24 h later, anti-IgG (mouse) or anti-Flag (mouse) was used for the pull-down. Both immunoprecipitation and whole-cell lysates were analysed by anti-Flag (NPC1), anti-STING and anti-tubulin. Data are representative of at least two independent experiments.
Extended Data Fig. 6 STING drives neuroinflammation in Npc1−/− mice.
a, Immunoblot analysis of the indicated proteins (left) in whole-brain lysates from BALB/c Npc1+/+, Npc1−/− and Npc1−/−Sting1−/− mice (n = 2). b, qRT–PCR analysis of the expression of ISGs in the cerebellum of BALB/c Npc1+/+, Npc1−/− and Npc1−/−Sting1−/− mice (n = 4). c, Heat map showing the expression of ISGs in BMDMs from BALB/c Npc1+/+, Npc1−/− and Npc1−/−Sting1−/− mice (n = 3). The mRNA expression level of each ISG was measured by qRT–PCR. b, Data are mean ± s.d. Unpaired two-tailed Student’s t-test. Data are representative of at least two independent experiments.
Extended Data Fig. 7 STING and IRF3, but not cGAS, are required for Npc1−/− disease pathogenesis.
a, Representative image for the body size of eight-week-old mice. b, Immunoblot analysis of proteins (left) in whole-brain lysates of mice of the indicated genotypes (C57BL/6J; n = 3). c, Heat map showing the expression of ISGs in BMDMs from mice of the indicated genotypes (C57BL/6J; n = 3). The mRNA expression level of each ISG was measured by qRT–PCR. d, Serum cytokine levels in four-week-old mice of the indicated genotypes measured by multiplex ELISA (n = 4). e, Heat map showing the expression of ISGs in Npc1+/+ and Npc1−/− BMDMs that were mock-treated or treated with the STING inhibitor C-176 (0.5 μM) or C-178 (0.5 μM) overnight (n = 2). The mRNA expression of each ISG was measured by qRT–PCR. d, Data are mean ± s.d. Unpaired two-tailed Student’s t-test. Data are representative of at least two independent experiments.
Extended Data Fig. 8 STING function in Purkinje cells and microglia.
a, Progression of Npc1−/− neuropathology. Fluorescent IHC staining of mouse cerebellum of Npc1+/+ and Npc1−/− mice at P7, four weeks old and eight weeks old. Calbindin is shown in red, CD68 is shown in green and DAPI is shown in blue. Representative images are shown (n = 3). Bottom panels are enlarged views. Scale bars, 200 μm (top); 30 μm (bottom). b, STING antibody validation for IHC. Fluorescent IHC staining with IgG (rabbit), anti-STING (rabbit) in wild-type C57BL/6J cerebellum or staining with anti-STING (rabbit) in Sting1−/− C57BL/6J cerebellum. Scale bars, 30 μm. Representative images are shown (n = 3). c, Heat map showing the baseline expression of ISGs in ex-vivo-cultured microglia isolated from P10–P12 mouse brains of the indicated genotypes (n = 3). The mRNA expression of each ISG was measured by qRT–PCR. Representative microscopy images of isolated microglia from indicated genotype of mice are shown at the bottom. Scale bars, 30 μm.
Extended Data Fig. 9 STING signalling activation in Npc1−/− and Npc2−/− cells and mice.
a, Immunoblot analysis of proteins (left) in whole-brain lysates of BALB/c mice of the indicated genotypes (n = 3). b, qRT–PCR analysis of cholesterol-synthesis gene (Hmgcr) expression in the cerebellums of mice of the indicated genotypes (BALB/c) (n = 3). c, Heat map showing the expression of ISGs in BALB/c Npc1+/+, Npc1−/− and Npc2−/− mouse cerebellum (n = 3). The mRNA expression of each ISG was measured by qRT–PCR. d, qRT–PCR analysis of the expression of some ISGs in the cerebellum of mice of the indicated genotypes (BALB/c) (n = 3). e, Immunoblot analysis of the STING signalling cascade. BALB/c Npc1+/+, Npc1−/− and Npc2−/− mouse BMDMs were stimulated with DMXAA (50 μg ml−1) for 0, 2 or 4 h. Phosphorylated and total proteins blotted are shown on the left. f, qRT–PCR analysis of the baseline expression of ISGs in Npc2WT, Npc2KD and Npc2KDSting1KD MEFs (n = 3). g, qRT–PCR analysis of the baseline expression of ISGs in BALB/c Npc2+/+ and Npc2−/− mouse BMDMs that were mock-treated or treated with STING inhibitor C-176 (0.5 μM) overnight (n = 3). b, d, f, g, Data are mean ± s.d. Unpaired two-tailed Student’s t-test. Data are representative of at least two independent experiments.
Supplementary information
Supplementary Figure 1
This file contains Western blot full scans.
Supplementary Data
This file contains the STING-APEX2 TMT-MS raw data.
Rights and permissions
About this article
Cite this article
Chu, TT., Tu, X., Yang, K. et al. Tonic prime-boost of STING signalling mediates Niemann–Pick disease type C. Nature (2021). https://ift.tt/3xWDz3n
-
Received:
-
Accepted:
-
Published:
Comments
By submitting a comment you agree to abide by our Terms and Community Guidelines. If you find something abusive or that does not comply with our terms or guidelines please flag it as inappropriate.
"type" - Google News
July 21, 2021 at 11:53PM
https://ift.tt/3eHp0tf
Tonic prime-boost of STING signalling mediates Niemann–Pick disease type C - Nature.com
"type" - Google News
https://ift.tt/2WhN8Zg
https://ift.tt/2YrjQdq
Bagikan Berita Ini
0 Response to "Tonic prime-boost of STING signalling mediates Niemann–Pick disease type C - Nature.com"
Post a Comment